AEROSPACE ASSEMBLY
CONTACT
Austin
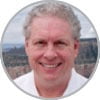
Lightweight battery-powered aircraft are ready for take-off.
Mitsubishi Automates Boeing 777 Fuselage Production
Austin Weber // Senior Editor // webera@bnpmedia.com
Mitsubishi Heavy Industries supplies the fuselage panels of the Boeing 777 jetliner. Photo courtesy Boeing Commercial Airplanes
The Boeing 777 jetliner is the backbone of many international airlines. The reliable workhorse, which has been used on long-haul flights for three decades, is produced in several variants. The aircraft’s 20-foot-wide aluminum fuselages range anywhere from 209 to 242 feet long.
Mitsubishi Heavy Industries Ltd. (MHI) assembles 777 fuselage panels in Hiroshima, Japan, and ships them to Boeing’s wide-body aircraft factory in Everett, WA. To improve productivity and boost quality, the airframer recently installed an automated fastening system supplied by Broetje-Automation GmbH.
“[The system is] one of the most advanced production lines in the aerospace industry,” claims Jonas Wermter, key account manager at Broetje. “The digitally interconnected and highly flexible panel assembly facility sets new standards in terms of quality and efficiency.”
Two state-of-the-art production lines include nine major fastening systems that improve flexibility and throughput. The goal of the multi year project was to create an automated assembly system that can quickly adapt to production fluctuations and cost reductions.
A flow line concept enables MHI to assemble multiple types of panels in different sizes and shapes on the same line, while significantly improving throughput and quality.
Traditionally, the aerospace industry has been slow to automate. “[That’s because manufacturers demand extremely accurate levels] of precision and quality,” says Wermter. “Commercial aircraft are large, complex products.
“The total number of planes produced annually is also significantly low compared to other manufacturing sectors, such as automotive or consumer goods,” explains Wermter. “Only a small part of the entire production process is automated.
“Due to complex processes [and tight tolerances], it’s often necessary to combine automatic and manual work in one workstation,” says Wermter. “Automation of entire lines is [rare] in the aerospace sector. However, new digital technologies, human-machine collaboration and Industry 4.0 [tools] are changing that scenario.”
Automation Challenges
Boeing 777 fuselage panels are produced at a world-class factory in Hiroshima, Japan. Photo courtesy Broetje-Automation GmbH
According to Wermter, aerospace engineers face several challenges when automating aircraft fuselage assembly applications. “A big challenge is the automatic correction of digital programs corresponding to the actual part in order to stay within the tight tolerances,” he points out. “Due to the large size and previous reliance on manual assembly steps, each part is slightly different to the digital file.
“Consequently, the machine needs to adjust its programming to the real shape of each part,” notes Wermter. “We use a comprehensive sensor system in the machines that feed into a digital twin to adapt the programming to each part.”
Another challenge is balancing the throughput of the automatic parts of the line together with manual fluctuations without slowing the line down. To address that, Broetje engineers developed a number of digital tools, such as the BA Job Control program of the line management system to support the dynamic management of the entire line.
Mitsubishi’s new automated production line features six multi-panel assembly cells that use state-of-the-art fastening technology. Photo courtesy Broetje-Automation GmbH
In addition, high forces within the fastening process occur during joining of inner and outer fuselages (skin assembly) on Broetje’s multi-panel assembly cells (MPACs). The machines can automatically rivet stringers, ribs, skin panels and shells.
“We refer to the part via BA-SCALE Positioning Sensors,” explains Wermter. “Then, the inner system (slave) follows the outer system (master), assuring a tight normality for the installation of Hi-Lok fasteners from the outside. The collar is taken on the shaft of the fastener from the inside, squeezing it on for final connection.
“For the fastening of the inner structures (frame-clip-assembly), we use one of our specially designed machines to drill and install the connections without losing the position within aircraft tolerances,” adds Wermter. “These tolerances are beyond the tolerances of standard robots. So, we use specially designed robotic systems and numerical control-based machines called a frame-clip assembly cell (FRAC) for this task.”
In the past, MHI used standard, stationary fastening machines. Instead of just adding extra capacity for the new program, the engineering team completely rethought the entire production line, including upstream and downstream process steps, as well as digital integration into the manufacturing execution system.
Three frame-clip assembly cells enable fast, high-precision assembly of the inner structure of the fuselage panels. Photo courtesy Broetje-Automation GmbH
This allows MHI to always have a streamlined production system that can be visualized in an MES via the line management system. All data from production can be monitored in real time by technicians and management. It also includes quality reporting and process analysis.
“This value far exceeds the technical requirements and underlines the excellent quality of the system,” claims Wermter.
The two new assembly lines incorporate six MPACs that use the latest fastening technologies such as automated lower tool changers and the newly developed active upper head squeeze process to dramatically reduce the shank gap. In addition, three FRACs complete the lines equipped with stacking axes for faster and high-precision assembly of the inner structure of the fuselage panels.
“The working level of these machines was lowered thanks to an innovative machine configuration that provides good accessibility and allows ergonomic maintenance,” says Wermter. “This concept [also] provides higher accuracy and rigidity compared to conventional industrial robotic systems.” This increases efficiency, while keeping operators safe and healthy.
Smart Factory Integration
The multi-panel assembly cell can automatically rivet stringers, ribs, skin panels and shells. Photo courtesy Broetje-Automation GmbH
A major improvement in throughput was achieved with the use of Broetje-Automation’s offline programming system (SOUL OLPS) and job control functionality.
“It allows [MHI engineers] to actively manage NC programs between different machines and according to the digital twin,” explains Wermter. “This makes programming and reactivity much more flexible and avoids unnecessary downtime for the machines. Combined with the line management system, it improves throughput.”
All fastening systems are digitally interconnected and fully integrated. Based on the combination of a digital twin, a full Siemens Sinumerik 840D integration and Broetje’s SOUL OLPS V55, a live simulation of all NC programs can be performed, even during the programming cycle.
Mitsubishi ships fuselage panels to Everett, WA, where the 777 jetliner is assembled. Photo courtesy Boeing Commercial Airplanes
“This speeds up programming,” says Wermter. “With real NC-machine data, it’s also possible to avoid [potential problems] and collision risks to keep the quality level high and production costs low.
“The factory of the future will be all about flexibility,” notes Wermter. “Each machine has to have the capability to digitally interact with the rest of the line and vice versa. This requires some software add-ons, but also a lot of hardware capability within each machine, such as the right sensor systems, computing power in the machine and the right management system.
“Additionally, the human-machine interface needs to enable [operators] to keep a full overview of the entire line,” explains Wermter. “One special function is the ability to swap NC programs between the different machines within the line. After one machine has started the execution of a panel, the line management system can swap or move the work to another machine, seamlessly executing the part where the previous machine has stopped.”
This enables smooth line flow and easier balancing between the machines. It also affects overall throughput.
“The digital MES integration of both lines with [our] line management system leads to a successful integration of the complex components of the assembly line toward the factory of the future,” says Wermter. “Exchangeable NC programs and detailed data collection ensures full visibility of each state of production in real time.
The SOUL job control software enables reorganization of the work orders for the machines via a digital interconnected backbone, if necessary. NC programs can be shared and redistributed between multiple machines to optimize throughput and overall equipment efficiency of the entire line. Digital twins also help improve operations at MHI’s Hiroshima factory.
“The biggest influence could be seen in speeding up the NC programming times,” Wermter points out. “Because we can use the real data of each part, programming is now up to four times faster for MHI. [With] upfront simulation and offline collision detection, there are almost no dry runs necessary any more. The reliability is much higher for both the machine and entire line.”
ASSEMBLY ONLINE
For more information on aerospace automation, visit www.assemblymag.com to read these articles: